What's the best use for our 'waste' biomass?
The ten biggest questions in energy & climate tech, Question 8
Current hypothesis: In an ideal world, probably pure carbon removal. In reality, who knows…
Before photovoltaics, there was photosynthesis
At first blush, photosynthesis seems like a delightfully elegant solution to our dual energy & carbon problems. After all, photosynthesis is nature’s favored mechanism for converting sunlight into chemical energy. We (animals) wouldn’t be here today if algae hadn’t first terraformed the planet by utilizing solar energy to soak up carbon dioxide from the atmosphere and replace it with an early form of air pollution: oxygen.
Photosynthesis artfully weaves all of the energy & carbon that it captures into plant matter, which makes up the bulk of what we call “biomass”… which is actually, by mass, mostly water. If you wring out the water, then you’re left with a “bone dry ton” of biomass, which is the most common unit of measure for the resource. Everything from wood chips, to sugarcane stalks, to cow dung can be normalized to a bone dry ton, although in reality “bone dry” is an aspirational goal. Most biomass is at least a little bit damp.
In any case, each bone dry (ish) ton of biomass contains roughly 10-15 million BTUs of chemical energy, mostly in the form of carbon-hydrogen bonds. That’s equivalent to 80-120 gallons of gasoline. (Like all fossil fuels, gasoline is itself a derivative of biomass: the result of eons of geologic pressure.)
Each bone dry ton of biomass also contains about half a ton of carbon extracted from the atmosphere. Because carbon dioxide weighs more than carbon, that actually represents 1.8 tons of carbon dioxide temporarily relieved of its problematic status as a greenhouse gas.
Each year, photosynthesis is responsible for an enormous global flow of energy & carbon. Land vegetation alone temporarily soaks up more than four times as much CO2 from the atmosphere as humans emit. Humans already lay claim to about a quarter of this annual flow to produce our food and fiber.
Alas… the vast, vast majority of all that carbon is returned to the atmosphere in short order. Nearly all of it cycles back into the atmosphere within about ten years, through either biodegradation or combustion.1 A trace amount is sequestered for a longer time in soil, but that’s nowhere near enough to counteract our emissions.
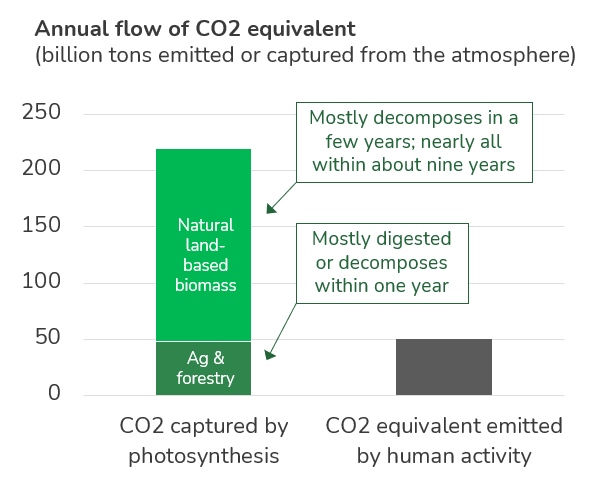
It’s impossible to overlook the untapped value in this massive global resource, which could hypothetically serve two civilizational purposes:
There are a multitude of ways to extract the energy embodied in biomass. For example, the simplest option is to burn it. There are also a lot of more complicated options, which mostly attempt to reconfigure the carbon & hydrogen atoms in biomass to more closely resemble fossil fuel molecules. Some of these options are pretty well established; others are just getting started. I’ll discuss them in more detail in a bit.
There are also a multitude of ways to sequester the carbon which biomass has already done the work of extracting from the atmosphere. The goal is to remove it from the natural carbon cycle for centuries to millenia. After all, fossil fuels were effectively created in this way, and were quite well sequestered underground until humans dug them up.
In fact, it’s possible to put any given dry ton of biomass to work for both of these purposes. There are several ways in which we might simultaneously extract useful energy from biomass and sequester its embodied carbon. These processes go by the general term of “Biomass Energy with Carbon Capture & Storage”, or BECCS. The simplest version entails burning biomass in a boiler to drive a steam turbine, and then applying carbon capture & geological sequestration to the resulting emissions. A more complex variant involves gassifying the biomass, separating out most of the hydrogen molecules to serve as an energy carrier, and sequestering the remaining carbon.
Regardless of the specifics, this dual value proposition tends to look extremely attractive on paper. BECCS is especially attractive to the kinds of optimization models that are currently used to plot out scenarios to achieve economy-wide decarbonization. Landmark studies like the Princeton Net Zero America Project tend to generate scenarios with as much BECCS as their model is allowed to include, given the scale of the available biomass resource… which is determined by the initial assumptions of the modelers.
This phenomenon naturally prompts an important initial question: How much of the world’s biomass resource can we realistically, sustainably, and ethically comandeer for BECCS, or for any other human activity?
Probably not much
Some people care about preserving natural ecosystems for moral reasons: e.g. prserving… well… Creation. (With a capital ‘C’, however you conceive of it.)
Everyone should care, I think, for purely selfish reasons. Nature currently provides us with all kinds of practically invaluable services, essentially for free, as long as we give it enough space to do so; for example: pollination, water purification, and erosion protection. Environmental economists refer to these sorts of things as “ecosystem services”, and there are many reasons to believe they’re at risk. Here’s a small selection of extremely worrying trends…
Deforestation in the Amazon rainforest, an enormous store of carbon, and font of biodiversity
70% population loss across thousands of monitored species, globally
Hence, in my view, we humans need to set aside any designs we might have on the vast majority of land that’s still growing biomass the natural way. We ought to let those plants photosynthesize and decompose according to their own rythyms. In fact, in my view, we probably need to start giving nature back some of the land that we’ve appropriated.
What about the land we’re already using for agriculture, grazing, and forestry?
The trouble is, most of the biomass that this land produces is already spoken for. We either eat it, wear it, or use it to build our homes; and in fact, we’re already putting some of it to use as a source of energy. About 7% of our global civilization’s primary energy supply still comes from what the IEA calls “traditional biomass”, which includes wood, peat, and dried animal dung. A tiny fraction comes from more ‘modern’ forms of biomass like corn ethanol.
So: Could we divert more of the productivity of our established agricultural & forest land into the energy sector? Maaaaaybe.
We could certainly tighten up our agricultural & food systems, in an effort to free up more land for crops dedicated to energy & carbon removal. The best way to do this would almost certainly be to eat less meat… or at least, to eat less meat produced by raising animals the old-fashioned way. Producing a single calorie of beef consumes around 30 calories of grain.2
Another option is “fake” or synthetic meat. I’m not particularly up to speed on the state of the art (despite being a big fan of Impossible burgers). But I’m hopeful that transitioning away from livestock-derived meat to these types of products could free up some more of our existing agricultural land. (This seems like a good topic for a future post…)
On the other hand, there are two big countervailing forces at play.
As the global poor keep becoming richer, my bet is that the world will demand more animal protein, not less. Many cultures around the world prize meat. And as difficult as technological change can be, I generally find cultural change to be even harder.
Studies tend to suggest that global warming will put significant pressure on agricultural yields, leading to greater risk of food insecurity. We’ll need to be very careful to make sure our agricultural system is resilient enough to feed 8-10 billion mouths as the risk of heat waves, drought, and flooding continues to rise. Personally, I believe we’re going to want to preserve scarce land, water, and fertilizer resources to grow “food, not fuel” (as the bumper sticker says).
Given these trends, productive land is on track to become an increasingly scarce resource. Today, land is still relatively cheap in the scheme of most large capital projects. In the future, access to productive land could easily become a dominant economic consideration. I personally believe that making more efficient use of land (and water, and nutrients) will be critical for our civilization to simultaneously manage the triple threat of climate change, ecosystem instability, and food scarcity.
This brings me back to photosynthesis, which is a shockingly inefficient means of converting solar radiation into useful energy. Nature’s marvels are still superior to human engineering in many domains, but harnessing photons is not one of them. In a given area, plants are able to convert 1-4% of incoming solar radiation into chemical energy. By contrast, commercial solar photovoltaics are able to convert 18-22% into electricity. Whether you want to consume that energy directly as electricity, or convert it to chemical energy via electrolysis, or use it to power a Direct Air Capture facility to remove carbon from the atmosphere, photovoltaics make much better use of land than photosynthesis.
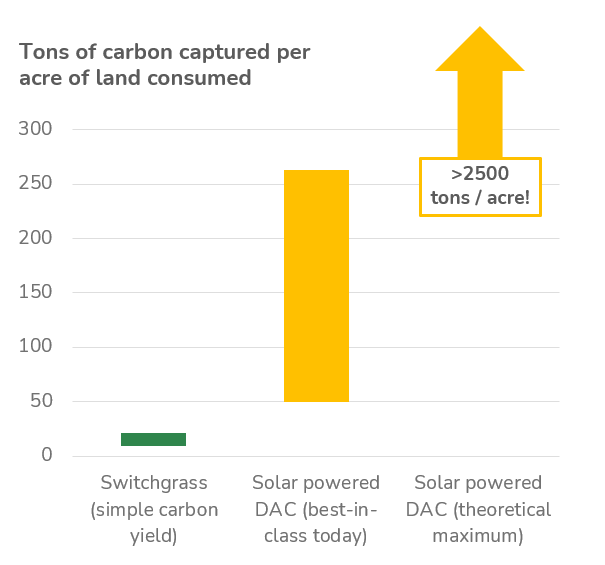
Ultimately, I’m not entirely opposed to dedicating additional land to growing crops for biofuel, or for carbon removal. However, my prior is to be very skeptical of those decarbonization strategies.
We’re left with “waste” biomass
There’s no such thing as “waste” in nature, but there certainly is in human agriculture, food systems, and forestry. For example…
We leave a whole lot of cornstalks and sugarcane fibers and other inedible woody biomass sitting on the ground after every harvest. The majority of the carbon in that biomass is either burned to clear the ground for the next crop, or else it decomposes back into CO2 within a few years. Many other crops also come with woody bits that we don’t have much use for, like oat hulls or pistachio shells. In some cases we end up finding some use for all this material, like bedding for livestock, but these tend to be fairly low value.
Whenever we harvest wood from forests, or saw logs into boards, we end up with twigs, and wood chips, and other thinnings. Just like agricultural waste, some of this is burned, while some is left to decompose.
In some forests, dead wood is no longer just “waste”. It’s a menace. In the US, Western states like California are actively seeking to clear out dead wood to reduce the risk of forest fires. One study found that there’s enough carbon removal potential in this kind of hazardous forest litter in California to offset nearly 5% of the state’s annual emissions.3
Our urban landscapes are filled with trees & hedges that need periodic trimming… and there’s only so much demand for mulch.
And of course, we also throw away a lot of food. This biomass tends to be especially moist, and tends to end up sitting in a landfill, which means it gradually decomposes anaerobically into methane. Because methane is a greenhouse gas about eighty times more potent than carbon-dioxide during its first twenty years in the atmosphere, this is an especially important waste problem to address.
Unfortunately, all of these sources of waste biomass share a number of common attributes which tend to make them difficult resources to work with…
Waste biomass is mostly comprised of woody, or “cellulosic” biomass. There’s a reason that animals which feed on cellulose all day, like cows, have multiple stomachs: it’s not an easy molecule to digest into the sugars & fats that fuel our bodies. For similar reasons, it’s also difficult to process cellulose into more useful liquid or gaseous fuels.
Waste biomass is mostly kind of wet, or at least damp. There’s a reason that the unit of measure the energy sector tends to apply to biomass resources is a “bone dry ton”. Bone dry is the way biomass is most efficient to process. For example, the simplest means of extracting useful energy from biomass is probably to burn it in a boiler; and everyone knows that wet logs make bad firewood.
Waste biomass is a highly distributed resource: scattered across millions of acres of farmland & forest, and thousands of processing facilities. The logistics required to collect enough of this resource to keep an industrial-scale facility churning are expensive.
Waste biomass is heterogeneous. Sure, at the molecular level, biomass is biomass: a bunch of carbon, hydrogen, and oxygen atoms with a handful of Ns, Ps, and Ks mixed in. But the specific form factor, moisture content, and purity of individual waste biomass streams is highly variable.
Waste biomass often comes with additional sustainability considerations. For example, how much “waste” agricultural biomass can we sustainably harvest from fields? It’s true that most of the carbon in that biomass ends up back in the atmosphere. But what about the carbon that makes its way into the soil? What about trace nutrients? In 2014, a landmark study of global bioenergy pointed out that there are still lots of questions we need to answer in order to make use of this kind of resource at scale…
All of these annoying features of waste biomass beg the question:
Is the juice worth the squeeze?
Is there really enough accessible waste biomass available to make it worthwhile to pursue?
Here are a couple of data points.
Today, in the US, we consume about 100 quads of primary energy, annually.4 The Department of Energy’s best estimate of the country’s untapped waste biomass resource is about 5 quads (give or take).
Globally, we emit about 50 gigatons of carbon-dioxide-equivalent greenhouse gases into the atmosphere. One recent, well-regarded estimate of the global waste biomass resource suggests that it amounts to about 2.5 gigatons of carbon removal potential per year.
So optimistically, if we were able to round up ALL THE WASTE BIOMASS, we could apply it to roughly 5% of our combined energy & carbon problems.
That’s a big number! So, yes, the juice could well be worth the squeeze.
Finally, this brings me to the question I initially set out to answer: Who ought to drink the juice? What’s the best use for all of our waste biomass?
Who wants to be a bio-millionaire?
It turns out, pretty much everybody.
These days, waste biomass is being considered by nearly every sector of the economy as a potentially low-cost element of a comprehensive decarbonization strategy. Here’s a partial list of the energy sectors hungrily eyeing this resource…
I. Power generation: As I noted above, the easiest way to harness energy from biomass is probably to burn it in a boiler. We already do this today, in some cases at dedicated biomass power plants, and in some cases as an amendment to coal-fired boilers. This activity is currently a rounding error in the scheme of our overall electricity supply.5 However, utilities are beginning to turn to biomass as a means of partially ‘repowering’ coal power plants with carbon neutral fuel.
Moreover, adding carbon capture to a biomass-fired boiler is also the simplest form of BECCS. It’s possible to use pretty much entirely off-the-shelf technology, but there are also companies like Arbor developing specialized, built-for-purpose solutions.
II. Natural gas: Within the natural gas business, local distribution companies are already ramping up their procurement of a form of bio-energy referred to as “renewable natural gas”, or RNG. Just like conventional natural gas, it’s mostly composed of methane, which means that with a little processing, it can be injected directly into existing natural gas pipeline infrastructure. This is a major benefit, because, as I’ve noted in previous posts, North American natural gas infrastructure is currently the largest continent-spanning energy delivery system in the world.
Most organic waste, when kept moist and deprived of oxygen, will eventually biodegrade into methane. Hence, capturing RNG from this type of environment does double duty for the climate. First, it prevents methane from leaking into the atmosphere; and second, it provides a drop-in fossil fuel substitute.
The easiest place to look for RNG is where anaerobic digestion occurs unprompted: namely, landfills, which are the source of about three quarters of today’s RNG supply. The next best places to look for RNG tend to be where we collect “pre-digested” biomass, meaning manure. This can be from livestock (typically concentrated in big, disgusting ponds), or from humans (in municipal waste treatment plants). In both cases, specialized anaerobic digestion equipment can accelerate the process. Development of these types of projects has surged in the past five years.
In the scheme of things, however, the supply of gas from landfills, cows, and human waste is relatively small. At best it might scale up to about half a percent of our total energy supply. In order to make a really big difference for the carbon intensity of pipeline gas, the industry will need to tap into the much bigger sources of waste biomass - namely, agriculture & forestry waste. Unfortunately, these biomass resources are nearly all comprised of cellulose, which is not so readily digested into RNG… or, for that matter, into any other gaseous or liquid fuel.
In order to convert cellulose into RNG, the most well-established process begins with biomass gasification. At a fundamental level, gasification technology is well-established, and has already been deployed at scale for the purpose of gasifying coal, primarily in China. Biomass gasification is a similar enough process that it can leverage most of the same principles and equipment. A few years ago, a company called SunGas Renewables was created to do just that by the Gas Technology Institute, a non-profit research organization sponsored by the gas industry.
Essentially, gasification plants ingest biomass and convert it into “syngas”, which is a gaseous blend primarily comprised of carbon monoxide & hydrogen. Thanks to decades of R&D in petrochemical refining, we now have a wide variety of established options for ‘upgrading’ those building blocks into practically any hydrocarbon molecules your heart may desire. For example, here’s how the Gas Technology Institute describes the process for converting syngas into RNG.6 (To be clear: I don’t actually recommend you read all this, but I’ve included it for illustrative affect.)
The gasification process converts wood waste to synthesis gas (or syngas) which is composed of hydrogen (H2) and carbon monoxide (CO) in a steam–oxygen atmosphere. An air separation unit (ASU) supplies oxygen (O2) and nitrogen (N2) to the gasification process. The dusty gas from the gasifier is sent to a tar destruction step carried out by a tar steam reformer where high-molecular-weight hydrocarbons are catalytically reformed into CO and H2 components, increasing the syngas quantity. The removal of the heavy tar component is required to avoid physical fouling of downstream equipment and catalysts that operate at low temperatures.
The hot, reformed syngas is cooled in gas coolers (heat recovery) generating superheated steam. The remaining dust in the syngas is removed in a filter unit. A water scrubber is utilized to further cool the syngas as well as remove the remaining contaminants in the gas stream. The raw syngas is slightly heated after scrubbing to reduce relative humidity before compression.
The compressed syngas is sent to Sour Shift where CO conversion into H2 and CO2 occurs in the presence of steam over sulfur-resistant Cobalt/Molybdenum catalyst. After the shift reactor, hydrogen sulfide (H2S) in the syngas is removed in the Acid Gas Removal (AGR) unit to produce a less than 1 ppm H2S-syngas stream. The presence of H2S can cause poisoning and deactivation of the methanation catalyst. Therefore, H2S removal is required upstream of the methanation unit. CO2 is also removed from the syngas in the AGR unit to meet the RNG quality specification after methanation. The concentrated stream of acid gases will be sent to a thermal oxidizer for complete conversion of hazardous components. The syngas leaving the AGR will pass through a Sulfur Guard, which removes the remaining H2S in the gas.
The CO, CO2, and H2 in the clean syngas are converted into CH4 in a 2-pass methanation reactor. The exothermic heat generated during the reaction is used to produce high-pressure (HP) steam. This will be combined with the high-pressure steam from heat recovery and will be sent to a steam turbine for power generation. RNG leaving the methanation unit will be dried in molecular sieve and silica gel beds prior to entering the pipeline.
The thing about gasification, though, is that it opens up all kinds of options beyond RNG. We know how to manufacture all kinds of drop-in fuels from syngas.
III. Shipping fuel: I mentioned Sungas Renewables above. The company’s first announced projects are not actually producing RNG; they’re producing methanol for the global shipping giant Maersk. Maersk, along with the rest of the shipping industry, views biogenic methanol as one of the most promising options among a short list of potential low-carbon shipping fuels. Relatedly…
IV. Aviation Fuel (currently going by “Sustainable Aviation Fuel”, or SAF): Airlines are currently desperate to get their hands on relatively low-cost, low-carbon fuels. When it comes to aviation, nothing but actual kerosene (jet fuel) will do without making major changes to basically every aspect of the airplane itself, and the airports it travels between. Biomass-derived fuels are more expensive than conventional jet fuel, but they’ll almost surely be cheaper than jet fuel produced via alternative “electrofuel” pathways (which I described in a prior post on clean hydrogen).
Jet fuel is a much more refined molecule than methane or methanol, so producing it from syngas requires more processing steps, but those steps are also well-established. Already, one of the largest players in the sustainable aviation fuel market is a company called Fulcrum BioEnergy, which is gasifying the organic (biomass) portion of municipal solid waste streams and upgrading to jet fuel. My firm Energy Impact Partners has invested in Metafuels, which has developed a proprietary process for efficiently increasing the share of jet fuel that can be derived from this sort of process.
V. Ground transport fuel: In the US, the ground transport market is already the largest consumer of bioenergy, in the form of biogenic ethanol blended into gasoline, which is required by the federal government. Ethanol is currently produced by fermentating the starch of corn kernels, which is a notoriously inefficient use of the crop, given that most of the biomass of a corn plant is embodied in the cellulosic stalk. Encouragingly, there are now a number of early-stage approaches to enable the efficient biodegradation of cellulose into ethanol (for example, Terragia), which would dramatically improve the sustainability of the ethanol business.
Of course, gasification is also an option. Syngas can be refined into gasoline or diesel in much the same way as it’s upgraded into jet fuel. In fact, the most well established process for producing hydrocarbons of any sort from syngas, the Fischer Tropsch reaction, yields a range of hydrocarbon fuels.
So, essentially, anyone whose business runs on any sort of liquid or gaseous fuel is eyeing biomass. And with current gasification technology, carbon-neutral or even carbon-negative biofuels of all kinds are within reach.
VI. BECCS, feat. Hydrogen: Astute readers will have noticed that one of the two major components of syngas is hydrogen, which is currently the bell of the ball in the worlds of climate policy & technology. One option for gasified biomass is to simply extract that hydrogen, and then capture & sequester the leftover carbon in the form of carbon dioxide.
This turns out to be the BECCS approach favored by many of the big, sophisticated net-zero models I described earlier. That’s because in realistic net-zero scenarios, carbon removal is always critical to balance out various stubborn sources of emissions that will inevitably linger well beyond the 2040s. Hence, given the choice to remove the carbon atoms in gasified biomass from the carbon cycle, or to ‘recycle’ them into a new hydrocarbon fuel, optimization models tend to opt for full removal. The fact that the remaining hydrogen can be put to use as a fuel, albeit an inconvenient one, is what makes this process such a theoretical winner.
On the other hand, gasification is expensive. Capturing and sequestering gaseous carbon dioxide is expensive. There have got to be cheaper ways to get rid of biogenic carbon.
Of course, there are, but they also dispense with the hydrogen.
VI. Pure carbon removal: Ultimately, the cheapest ways to remove biogenic carbon from the atmosphere all tend to involve burying it in some form. There are an increasing number of startup companies coming up with clever ways to do this while ensuring the carbon stays buried for a long, long time. For example, Graphyte is casting biomass into dense, solid blocks, then applying a coating to prevent microbial intrusion, and then burying them. Charm Industrial is pyrolyzing biomass into a dense oil, and injecting it deep underground (a poetic reversal of the oil extraction process we’ve perfected over the past century). And Carboniferous is burying biomass not in the ground, but deep in anoxic underwater basins.
These companies are all aiming to be paid solely for the service of carbon removal, and are aiming to do so for less than $100 per ton of carbon removed. (Graphyte claims it already is.)
Lastly, there’s another category of biogenic carbon removal solution which I tend to find compelling, but remains somewhat controversial, which is biochar.
Biochar is a carbon-rich substance which appears a lot like charcoal. It’s created through the process of pyrolysis, which entails heating biomass in a low oxygen environment. The resulting product is typically mixed into soil, in which it has two ostensible jobs: 1) Improving soil fertility, and 2) Keeping that carbon out of the atmosphere for at least a hundreds to thousands of years. In a carbon-constrained world, the latter job is almost certainly the much more valuable one.
There’s controvery on both fronts. As with any topic involving soil science, the truth seems to be as complicated, variable, and dynamic as the soil itself. When it comes to soil fertility, the particulars of climate and geology matter a lot; for example, biochar provides more well-substantiated benefits in the tropics than in temperate climates. When it comes to the crucial question of how long the carbon in biochar resists biodegradation, the error bars are very large. I’ll quote a big 2019 meta-study on the topic: “Estimation of [carbon] residence time under field conditions range from 6 to 5448 years”!7
And yet, I still can’t help but root for biochar. It’s probably the simplest possible mechanism for biomass-based carbon removal, and it has some entitlement to be the cheapest. Also, given the soil fertility and erosion challenges our civilization is facing, I really like the fact that biochar doesn’t remove carbon & trace nutrients from topsoil entirely (like
I’m especially intrigued by the approach taken by a company called Climate Robotics, which is producing biochar from agricultural waste right in the field, using mobile pyrolysis units, where it can immediately be applied to the soil. This approach addresses a big sticking point for essentially all other companies reliant on waste biomass: the daunting logistics of collecting enough of the stuff to keep an industrial-scale facility churning.
It’s also clear from academic research that not all biochar is created equal. One key variable is the ratio of carbon to oxygen in the product. Studies strongly suggest that biochar with high ratios of carbon to oxygen should last for over a thousand years in soil (for example8)… which is plenty of time for humanity to get through our current carbon predicament. Or not, in which case this will be a moot point!
VII. Composting & food waste upcycling: Food scraps make up a relatively small share of the overall waste biomass resource, but they play an outsized role for two reasons. First: today they’re mostly landfilled, which means they mostly degrade into methane, which will become either an especially potent contributor to global warming, or a highly valued source of RNG. Second: they’re the only category of waste biomass that just about everybody encounters on a daily basis, so they represent an especially prominent way for individuals to make a difference. Call me a sap, but I think that’s important.
Composting is probably the oldest way of dealing with food waste. The goal is to accelerate decomposition of waste to reduce odors and pests, and to generate a nutrient-rich soil amendment which can be mixed back into the earth to enhance fertility.
Unfortunately, attempting to quantify the impacts of compost on soil carbon formation is an even more maddeningly difficult activity than quantifying the impact of biochar. After all, biochar is a relatively standardized, inert carbon product; meanwhile each pile of compost is a diverse ecosystem comprised of living, breathing microorganisms continuously interacting with each other, the soil, and the air.
On the other hand, every home gardener knows that a good mix of compost absolutely improves soil fertility. And we wouldn’t be here today if the composting process didn’t lead to the gradual accumulation of durable soil carbon. Worldwide, there’s over 200 times more carbon stored in topsoil than humans emit every year. If we could only restore the topsoil we’ve lost since the beginning of human civilization, we’d negate about ten years of human greenhouse gas emissions.
On the other hand, according to a recent study by the US Environmental Protection Agency, it turns out that the best use for food waste is probably to reprocess it into animal feed. Recycling embodied energy & nutrients in this way reduces all of the emissions associated with growing new feed from scratch.
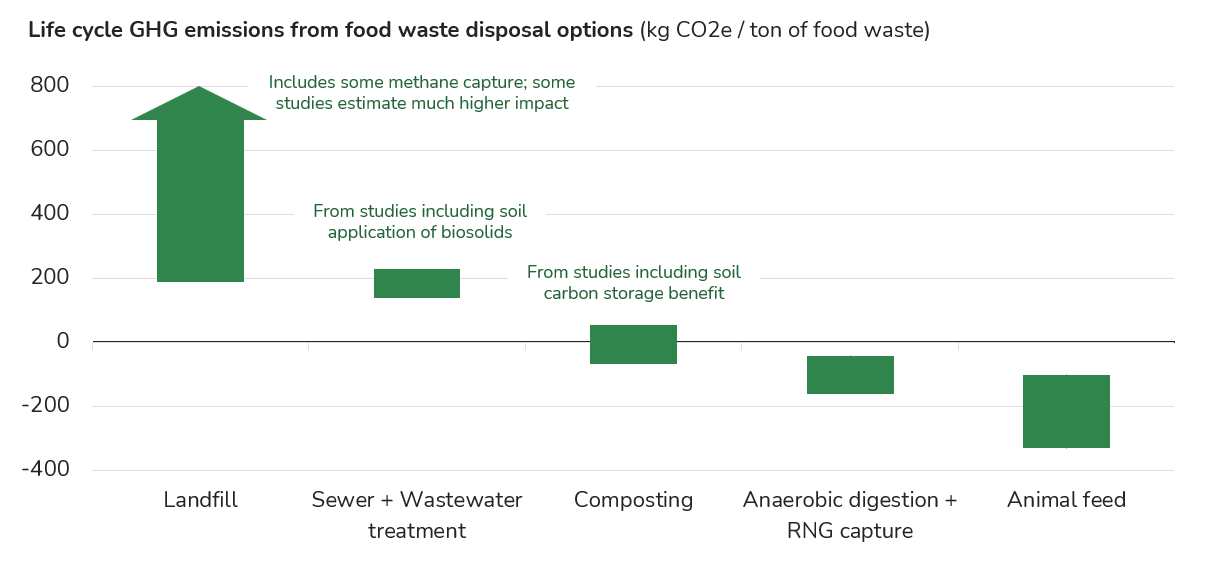
At Energy Impact Partners, this kind of analysis led us to invest in Mill.
Mill makes a clever little bin that consumes a small amount of electricity in order to convert food waste into what they call “Food Grounds”, a dry material which is easy to ship to a centralized facility where it can be processed into animal feed.
The way it currently works is: 1) You sign up; 2) Mill ships you a bin; 3) You dump your food waste in it; 4) Every few weeks you ship the grounds to Mill in a prepaid box; and finally 5) Mill processes the grounds and upcycles them into chicken feed. You get to know that your waste is actually reducing emissions (especially relative to a typical landfill scenario). Also, your garbage will stop stinking, and if you’re in a place where you pay by the trash bag, you’ll save a little money. Here’s what the Mill bin looks like in a really nice kitchen:
VIII, IX, X, etc. The list goes on. Low-carbon concrete solutions, like CarbonBuilt, are pursuing waste biomass as a combined source of energy and carbon-dioxide for novel curing processes. A variety of bioplastic companies want to turn waste biomass into packaging material. You get the idea.
So which is the BEST use for waste biomass?
Here’s the economist’s answer: The best use is the one whose market value most exceeds it’s cost… given a rational, universally-applied carbon price, of course.
In that case, here’s my best comparison of the leading contenders for utilizing the most abundant resources, agricultural and forestry waste.
The clear winner is pure carbon removal, because it’s valuable and cheap. In fact, at this fundamental economic level, I’m not convinced that any of the other other options make sense at all.
RNG is probably the cheapest fuel to produce through biomass gasification, because methane is the simplest hydrocarbon to assemble from syngas. However, RNG is simply not that valuable, because natural gas has such a low cost to begin with, as well as a relatively low carbon intensity. Even a carbon price of $200/ton is not currently enough to make cellulosic RNG economically sensible.
Jet fuel is much more valuable than methane, but it’s also, much more expensive to produce because it requires several additional, expensive processsing steps. The numbers don’t add up, even with a $200/ton carbon price. However, this is a space in which new technology can probably make a big difference. Substantially better technology for upgrading basic organic building blocks into jet fuel, such as Metafuels’ selective catalytic solution, could cut the cost of production by more than half.
BECCS: Producing hydrogen while capturing and sequestering the leftover carbon, the darling of net-zero models everywhere, is both valuable and expensive. Admittedly, I have less visibility into the cost of this pathway. However, I’ve seen a few real world data points from folks in the early stages of trying to pull this off. There’s a long way to go before this variant of BECCS lives up to its theoretical promise.
Do these economic fundamentals really matter in the real world?
Today, probably not so much.
On the demand side of the market, biofuel buyers appear to be racing to lock in supply at nearly any cost. Airlines are buying up SAF with no obvious imminent carbon price exposure. A number of gas utilities are doing the same for RNG. Meanwhile, early buyers of carbon removal credits are willing to pay even more exorbitant prices.
In short, in case you didn’t already know: There’s no such thing as a rational, universally-applied carbon price.
On the supply side of the market, this sense of urgency is beginning to entice more project developers into the fray. It’s possible that we’re seeing the first signs of a run on waste biomass resources. Sure, there’s plenty of the stuff out there, but the logistics of sourcing and transporting it can be very tricky. Plus, in order to access this resource at all, you need to talk to the people who control it.
They’re at the foundation of this nascent market; the farmers, foresters, food processors, and other entities sitting on piles of previously low-value cellulose. I don’t personally know this lot well, but my understanding is that they don’t tend to have much spare time to research the comparative cost of various carbon removal techniques. At least for now, if a Biomass-to-X project developer approaches them with a minimally viable offer to take some waste off their hands, they might just accept.
In sum: this is many years from being a market with sophisticated price discovery mechanisms.
Let a million (rotting) flowers bloom
It’s probably for the best that the waste biomass market is still so messy. At this early stage, I’m glad to see so much experiementation across all sorts of different strategies for utilizing such a large & untapped resource.
We could be sitting on an absolute treasure trove of waste. I know this is a cop out answer, but ultimately we need a lot more experimentation to figure out the best way to use it.
Please check out the rest of the Ten Biggest Questions in Energy & Climate Tech. And also, please subscribe!
Combustion: e.g. in a wildfire, or a controlled agricultural burn
“Energy and protein feed-to-food conversion efficiencies in the US and potential food security gains from dietary changes,” A Shepon, et al, Environmental Research Letters 11, 2016
”Getting to Neutral: Options for Negative Carbon Emissions in California”, Baker et al, Lawrence Livermore National Laboratory, Aug 2020
“Quads” = Quadrillion BTUs
In the US, biomass generated about 1.2% of electricity in 2022.
“Low carbon renewable natural gas from wood wastes”, Gas Technology Institute, Feb 2019
“Potentials, Limitations, Co-Benefits, and Trade-Offs of Biochar Applications to Soils for Climate Change Mitigation”, Tisserant and Cherubini, Land, 2019.
“Review of the stability of biochar in soils: predictability of O:C molar ratios”, Spokas, Carbon Management, 2010