Will CCS make a lot of these other questions moot?
The ten biggest questions in energy & climate tech, Question 10
Current hypothesis: Nope. But it could be a regionally important solution.
One way to think about the world’s greenhouse gas problem is that it’s just another type of waste problem.
All of my prior posts in this series of big questions about how to deal with carbon ‘waste’ have explored how we might stop producing it in the first place, mostly by burning a lot less fossil fuel. This strategy requires finding viable alternatives to fossil fuel, both as a primary energy resource and as an energy carrier. I’ve spent the past eighteen months writing about lots of promising solutions… but also, why these solutions are not yet fully sufficient to get the job done. In many sectors, replacing fossil fuel while maintaining energy affordability & security is, I’m sorry to say, wickedly difficult. The energy trilemma is real.
But the thing is… We don’t actually need to replace fossil fuel in order to address our carbon waste problem. Nor do we need to stop producing carbon waste; we just need to keep that waste from accumulating in the atmosphere and oceans. Ceasing to waste is not really how we, humans, manage our other big waste problems. Instead, for better or for worse, we tend to deal with our waste by isolating it, treating it (if we must), and dumping it somewhere that it won’t cause much harm.
For example, take the original form of human waste: human… uh …waste. Given that excrement is an essential part of human life, our only choice to avoid wallowing in it has been to follow the playbook I outlined above. As soon as we figured out the basics of sewage systems, we began laying extensive underground networks of pipes, at considerable cost, as quickly as we could. Modern sewer systems were not invented until the 1850s, but adoption was very rapid in densely populated areas, like Brooklyn, New York. Here’s a map of the Brooklyn sewer system as of 1902.
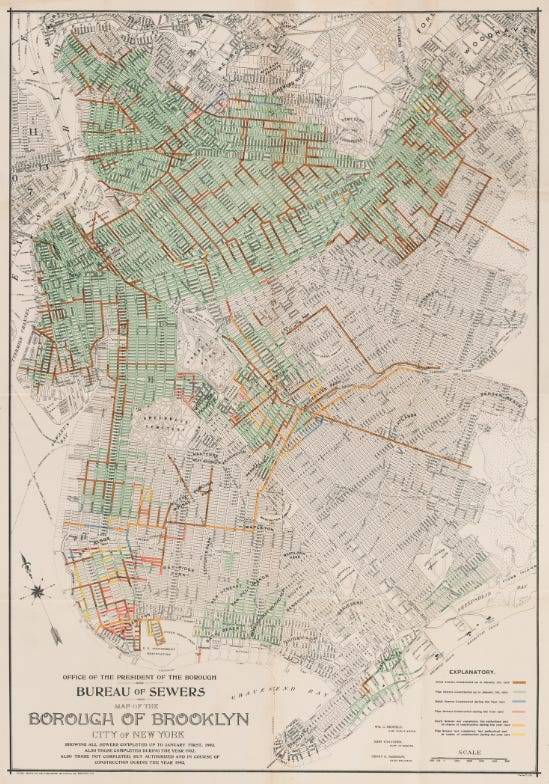
This was no small feat! Installing pipes requires digging up streets, which is tremendously expensive and disruptive. Operating & maintaining sewer systems also takes a lot of ongoing work: US municipalities spent over $60b on sewer systems in 2020.1 And we spend about the same amount on managing our other primary form of garbage: municipal solid waste. All the stuff we generally refer to as plain old “trash” costs Americans another $70b each year to landfill or recycle2.
This means we’re already spending around half a percent of GDP on these two big waste streams alone! The question is: How much more expensive would it be to deal with carbon in a similar manner? (Note: I’m definitely not the first person to pose this question…3)
This is the basic idea of CCS (Carbon Capture & Sequestration): Round up a bunch of CO2, then pump it into deep underground into a geological formation in which it can be contained in perpetuity.
Consider a thought experiment
For the sake of discussion, assume that the US decided to deal with a third of our total carbon dioxide emissions using CCS. For the moment, assume that the total cost of capturing carbon, injecting it into a pipeline, and then sequestering it securely underground sums up to roughly $150 per ton of CO2. For now, take my word for it that $150 per ton is an optimistic, yet very reasonable number. (I’ll return to cost in a bit.)
How much would this new form of waste management cost the United States?
Well, in one sense it would be pretty darn expensive: roughly double what it costs to manage our two other biggest waste streams combined: ~1% of GDP.
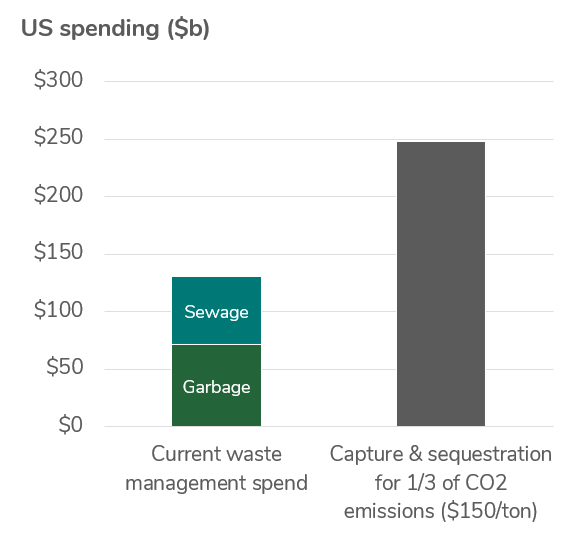
In a broader sense, however, 1% of GDP is not such a big deal. In the context of historical energy price variability, 1% of GDP is practically noise.
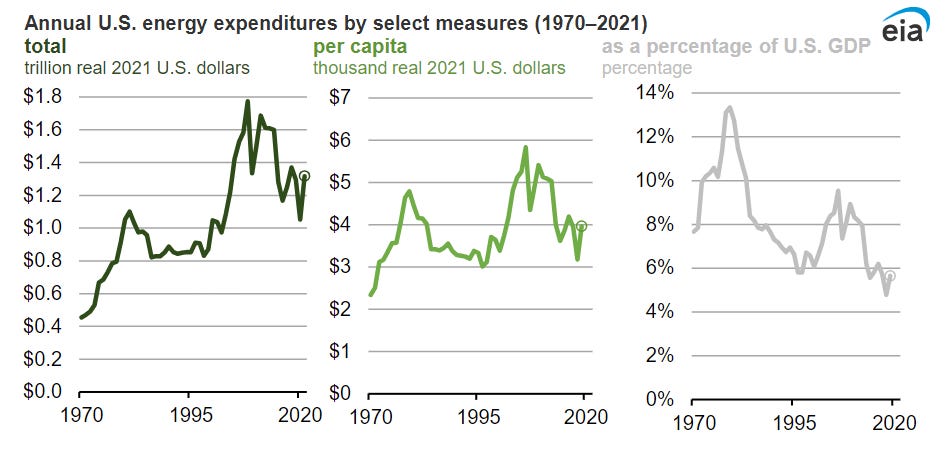
Adding this cost to our national energy budget tody would still leave us with a lower energy cost burden than we’ve managed to live with for the majority of the past five decades.
So theoretically, I think we could apply CCS to a substantial share of our carbon problem without breaking the economy.
(And yes, this hypothetical is applicable to the cost of carbon emissions abatement in general, not just in relation to CCS; but I find that the ultra-broad applicability of CCS makes this mode of thinking especially revelatory.)
First, a primer on how CCS works
There are very few places in which CO2 can be found in a pure, concentrated state. Mostly, CO2 is interspersed with other gasses, either in the emissions from a boiler, turbine, or engine… or simply floating around in the ambient atmosphere. Hence, capturing it really means separating and isolating it from these other gases. In general, there are two different, fairly well-established methods for separating gas molecules.
Membranes which only allow particular gases to pass through.
Materials which selectively bind with certain molecules in particular environmental conditions and then release those molecules under different conditions.
When it comes to capturing CO2, the latter approach is by far the most technically proven in real field conditions. The vast majority of active carbon capture projects to date employ a class of liquid solvents called “amines” which can be rapidly cycled through sequences of binding and releasing CO2. This process requires two big tanks full of the stuff. In the first tank, a stream of gas containing CO2 flows through free amines which readily grab onto CO2 molecules. These bound amines are then pumped to the other tank, in which treatment with steam causes them to release the CO2 they’re carrying. The pure CO2 is effectively captured, and the amines are cycled back to the first tank to begin the process again.
In the scheme of climate technology, amine capture currently sits in a somewhat awkward, liminal zone between “technically proven” and “commercially ready”. The process fundamentally works, and it can be scaled up to address nearly any large point source of emissions. However, even the most well-established amine processes have yet to be demonstrated with great success at industrial facilities or power plants.
So far, there have only been two commercial demonstrations of amine capture systems at power-plant-scale in North America: the Boundary Dam project in Saskatchewan, and the Petra Nova project in Texas. Both were initially cheered by the industry when they were retrofitted for CCS (in 2014 and 2017, respectively). Both sought to offset the cost of CCS by selling CO2 to oil producers, who can make use of the pressurized fluid to force more oil out of otherwise declining wells. (This form of “enhanced oil recovery”, or “EOR”, is obviously not the most climate-conscious way to dispose of CO2, but it’s a good way to pay the bills in the absence of a price on carbon.) Unfortunately, both of these projects struggled to achieve critical operational goals. Petra Nova was forced offline in 2020 amidst falling oil prices, and sat idle until late last year. Boundary Dam was also taken down for a long stretch in 2021 due to mechanical failures.
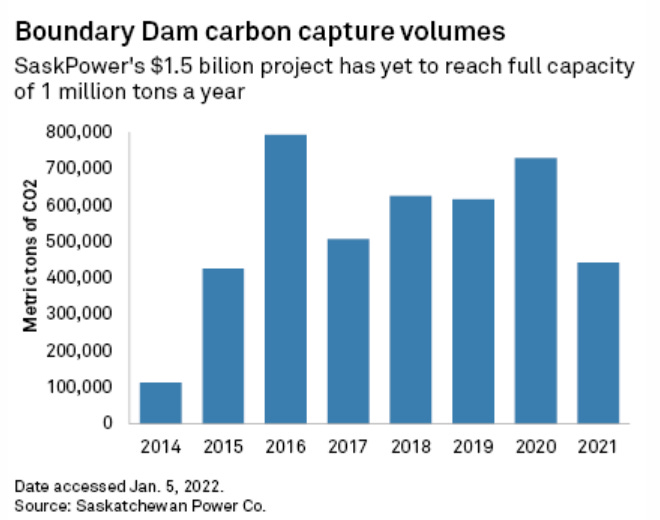
Clearly, there are still lots of kinks that need to be worked out in order to make amine capture successful. Yet even if we’re able to resolve the issues exhibited by early projects, this approach to CCS suffers from some fundamental drawbacks. Most importantly, the scale of amine tanks, pumps, and other sub-systems required to capture high levels of CO2 is absolutely massive. There is just no way around the immense capital expenditures required to build a huge chemical plant. Moreover, attempting to design a system to capture an ultra-high share of the carbon emissions from a facility requires an especially large volume of amines. Theoretically, 90-95% capture ought to be possible, but the case of Boundary Dam illustrates that such high capture levels are not so easy to achieve in practice. It’s probably inevitable that these systems will allow some carbon to be emitted.
There are also a number of much less well demonstrated approaches to capturing carbon, and a slew of companies working on them. Some are aiming for incremental improvement by tinkering with amine formulations and system design; while others are experimenting with entirely different classes of material, such as solid sorbents or membranes. One of our portfolio companies at EIP, Carbon America, is developing a cryogenic process to effectively freeze the CO2 out of an emissions stream. This process should be able to handle flue gas with a relatively low concentration of CO2 and high levels of other contaminants, without requiring such an intimidating capital outlay as an amine system.
(I’m proud to say that the Carbon America team has now completed the largest cryogenic capture pilot in history, at the US National Carbon Capture Center (operated by EIP partner Southern company). The project captured greater than 90% (sometimes up to 99%) of the CO2 from a flue gas stream with a concentration of less than 5%. In other words: this is the real deal. I think it could be transformational.)
In sum: There’s very little question that carbon capture can work, but there are lots of remaining questions about how well it will work, which technology will work best, and most importantly how much it will cost.
What about the ‘S’?
So you’ve gone and captured some carbon…
Now you need to do something to keep it out of the atmosphere in perpetuity; or at least, in my view, for a few hundred years, while we sort out the most acute phase of our civilization’s greenhouse gas problem.
I believe the only scalable strategy is to sequester it underground.
Okay, okay… There are also some other strategies worth considering. Climate tech junkies often add a ‘U’ in between the ‘CC’ and the ‘S’, which stands for “utilization”. I’ve already described the most mature form of CO2 utilization, which is enhanced oil recovery; in fact, EOR has been critical for the economic viability of nearly every carbon capture project to date. However, EOR is probably not compatible with deep levels of decarbonization.4
The most exciting set of utilization alternatives all involve putting CO2 to work as an ingredient in durable materials. Theoretically, heavy construction materials like cement, concrete, and asphalt might be able to serve as repositories for a gigaton or two per year, making utilization a meaningful alternative to sequestration. But I’ll admit that I tend to be pretty skeptical of how scalable these approaches will be in practice. (That’s a topic for another post.) For now, suffice to say I believe that making CC will require an enormous amount of S in order to make a meaningful dent in carbon emissions… even if some U’s turn out to be commercially interesting.
So: Is there enough room underground to store an enormous amount of carbon? You bet there is.
The consensus among geologists is that there’s enough space in naturally occurring formations to sequester many thousands of gigatons of CO2 around the world. For context, the US currently emits around 6-7 gigatons of CO2 per year, and the US Geologic Survey estimates that we have room for approximately 3,000 gigatons underground.
Currently, the ideal geologic formations for sequestration are saline aquifers, as they’re filled with what the industry calls “pore space” for stashing CO2. But, there are also a handful of companies pioneering new techniques for trapping, and even mineralizing CO2 in other kinds of formations. (In other words: they’re aiming to bind CO2 with other elements which transform it from a pesky gas into a rock. This would certainly address concerns about the longevity of carbon sequestration!)
All in all, it’s definitely easier to locate great sequestration sites in some regions than others, but these are not especially rare finds. Sites which geologists consider “good enough” are pretty well distributed across the world.
On the other hand… this massive storage resource is not so simple to access in practice as it is in theory. To understand why, consider this illustrative, five part plan for developing a large sequestration site:
First, obtain the legal right to access all of the pore space into which your carbon might flow. Note that CO2 is probably not going to behave exactly as you’d like it to, once you start pumping it underground. There’s a risk that it seeps out of your pore space and into your neighbor’s, who might not be thrilled with the knowledge that the earth below their property is filling up with CO2.
Find the right spots, and drill some holes in the ground. Fortunately, the oil & gas industry is already pretty darn good at this, which probably makes this the least problematic step in the plan.
Next, pump the CO2 that you’ve captured into those holes. Of course, your emitting facility may not be not located right on top of your sequestration site, so this step probably requires building a pipeline to transport gaseous CO2. In some cases it may necessitate a very large pipeline spanning tens of miles. (Maybe hundreds?)
Then comes the most technically challenging part: actually pumping massive amounts of CO2 underground. Yes, the oil & gas industry has some experience doing this in the context of EOR; but there is still substantial uncertainty regarding how difficult and expensive the process will be in other kinds of geologic formations. Frankly, big picture studies assessing the opportunity for CCS tend to gloss over this step; but conversations with real world operators have left me concerned that the risk involved in this step is underappreciated.
Lastly, in order to make sure that all the carbon stays buried, somebody needs to be responsible for maintaining all this new infrastructure, effectively in perpetuity. This is not yet a well understood liability… which is not the sort of thing insurance companies and investors like to see on a balance sheet.
And through all of these steps, CCS developers need to navigate the same kinds of permitting challenges holding back other forms of energy infrastructure. Permitting authorities have very little experience in this area, and the familiar forces of “Not In My Backyard” have already quashed some of the first big, ambitious carbon infrastructure projects under development in the US. It turns out that electric transmission is not the only form of energy infrastructure staring down the barrel of “NIMBY” and “BANANA” resistance. (BANANA = “Build Absolutely Nothing Anywhere Near Anybody.”)
Given these daunting hurdles, it’s easy to be pessimistic. But I’d rather offer Steel For Fuel readers an optimistic vision for the future of CCS infrastructure. Back in 2020, a think tank called the Great Plains Institute published exactly that: a measured, yet ambitious vision of a robust network of CO2 pipelines and sequestration sites spanning the US heartland.
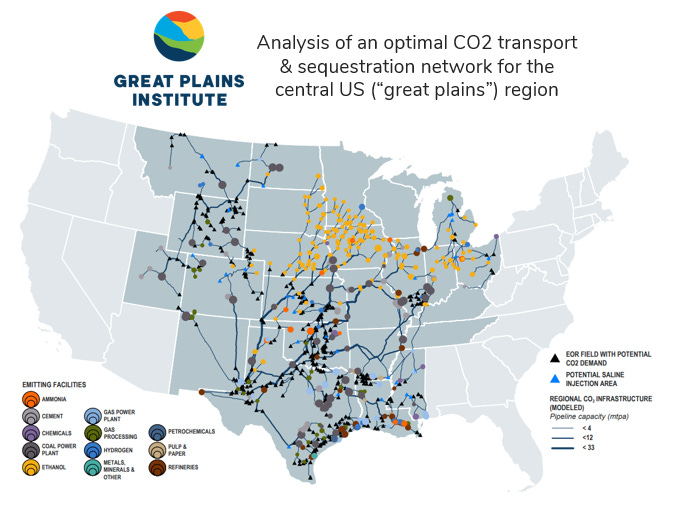
Ultimately, building a network like this is almost certainly going to be critical for any plan in which CCS plays a major role in decarbonization. The cost of CO2 transport & sequestration, for an individual project going it alone, can be as much as $30-50 per ton of CO2. But the cost of sequestration for projects in a large network could fall as low as single digit dollars per ton. Hence, CCS really does necessitate a new form of regional (or even national) infrastructure… not so different from those sewer pipes running beneath our cities today.
Speaking of cost…
There’s a famous quote by an early 20th century bank robber named Willie Sutton. When the FBI finally caught Sutton, and asked him why he was robbing banks, Sutton replied “Because that’s where the money is.” In other words: Banks contain very large, concentrated piles of money. For similar reasons, CCS is theoretically easiest to deploy where there are very large, concentrated sources of CO2.
Carbon capture is inherently a chemistry problem, and chemical processes tend to benefit tremendously from economies of scale. In fact, the benefits of scale in the chemical industry tend to be so predictable that engineers have come up with a rule of thumb, called the “Six Tenths Rule”, which asserts that increasing the capacity of a plant by 10X will only increase the cost of the plant by about 6X. (Thanks to my EIP colleage Greg Thiel for lodging that heuristic in my brain.) Additionally, as I just discussed, transporting and sequestering CO2 is generally going to be much cheaper when it’s done at very large scale.
CO2 concentration is also an important variable, and this one is based on thermodynamic fundamentals. In layman’s terms: The universe tends to prefer things like molecules to be evenly distributed, everywhere. Hence, gathering up CO2 takes a lot of work, and CCS is inherently easier to apply to a stream of CO2 that’s already in a relatively concentrated state. For context…
The concentration of CO2 in the earth’s atmosphere is about 420 parts per million, or 0.04%.
The concentration emitted by a natural gas fired power plant is in the range of 4-6%.
And the concentration emitted by a coal power plant is 12-15%.
These two variables - scale and concentration - are almost surely going to govern the cost of CCS regardless of the specific capture technology employed. There’s a reason why sites like Boundary Dam and Petra Nova have been viewed as important test beds for CCS, and why the struggles of these early projects were so damaging to the industry’s reputation: coal power plants are some of the largest sources of CO2, with some of the highest concentration emissions one can find. If CCS can’t make it in coal power… it’s reasonable to be skeptical that it can make it anywhere.
On the other hand, perhaps starting with such enormous projects was a mistake. There are a few classes of industrial facilities which are nowhere near as big as coal power plants, but more than make up for their small scale with ultra-high CO2 concentration. For example, natural gas processing facilities are purpose-built to separate CO2 and other contaminants from methane, which means they’re practically doing most of the hard work of carbon capture already. (In fact, some of these sites have already been tapped, for over a decade, to supply CO2 for EOR.) Another option is ethanol plants, which yield highly concentrated CO2 from the process of fermentation. It’s no surprise that these facilities are currently seen as the lowest-hanging fruit by the resurgent CCS industry.
Of course, there are all kinds of other variables which determine how much it might cost to capture CO2 from any given facility. For example: there’s the layout of a plant, the space available for capture equipment, and the distance from a viable sequestration site. There’s also still a lot of uncertainty simply because of the early stage of technology deployment. Hence, estimates of the cost of CCS tend to come with pretty big error bars. Here are some recent ranges estimated by the US Department of Energy:
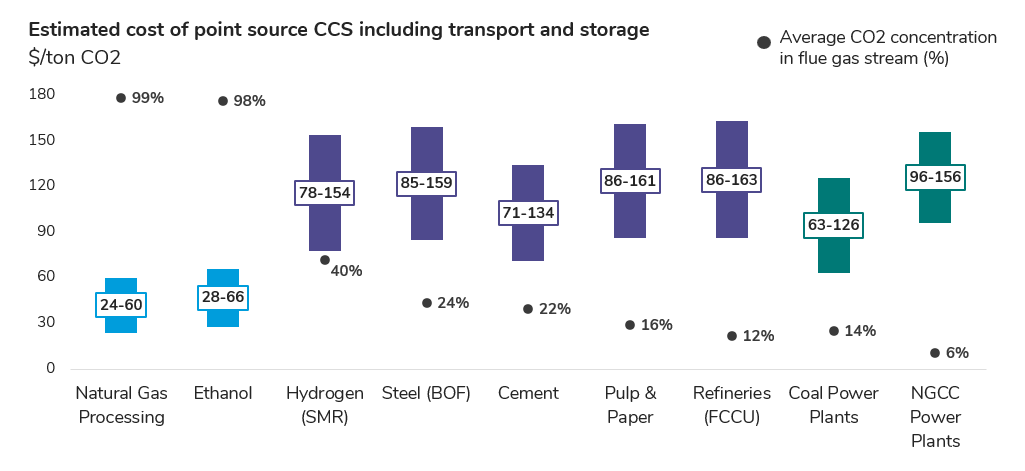
Unfortunately, this high degree of uncertainty has begun to act as a major drag on the CCS industry. The risk of cost escalation is now probably the biggest factor holding back the next generation of projects. In fact, many developers (and financiers) are concerned that costs could spiral even higher than the DOE’s ranges.
On the other hand: Remember my little thought experiment from the beginning of this post? The price I used as a placeholder for the cost of CCS was $150 per ton, which is near the high end of all of these estimates. Hence, if the combined forces of innovation and learning curves can bring the cost of CCS down into these ranges, then we could theoretically apply CCS to many big “point sources” of emissions without causing crippling energy price inflation.
But will we?
That depends on the alternatives.
In general, as I’ve discussed in many prior posts, renewable power is now the alternative to beat when it comes to clean energy supply in many parts of the world. That’s increasingly true even when you factor in the added cost of energy storage for delivering clean power with high availability, and the assurance of firm capacity during peak hours.
However, renewable power is currently going through a number of major growing pains. (For a somewhat depressing detour, check out my recent post, “Adolescence for renewables, Retirement for Oil”.) Sadly, these trends are making me somewhat less confident in wind & solar power’s ascendance. Moreover, there are some regions in which renewable energy was never on track to be all that cost competitive - e.g. anywhere that has been banking on lots of offshore wind, or planning to import renewable energy from afar in the form of ammonia. In these places, like the Northeastern US or the entirety of Japan, my view is that nuclear energy is probably going to be a much better option. It’s been about a year since I first began predicting a global nuclear renaissance, and frankly, I now find myself with even greater conviction in its inevitability.
So, how does CCS stack up to these alternatives? Of course, the answer is complicated. I’ve tried to summarize some of the economic fundamentals in this chart, but there’s no way to incorporate all of the nuances which will determine the real world competitive dynamics between clean power generation and CCS.
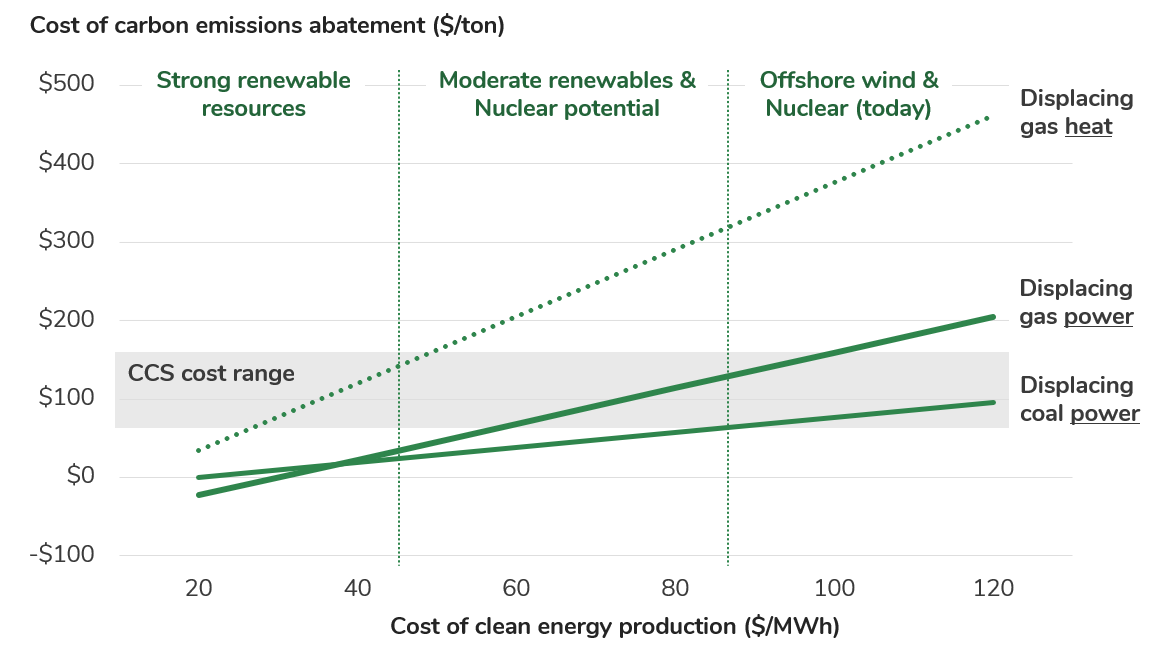
Consider the case of coal power generation:
Despite recent inflation in renewable power prices, I still believe that solar & wind power are on track to beat coal power generation with CCS in most places. Coal combustion emits so much CO2 that the advantage of relatively high CO2 concentration is outweighed by the sheer magitude of carbon that need to be captured. This is especially true in North America & Europe, where coal generation is weighed down by other environmental burdens, and the advanced age of the fleet makes massive CCS retrofits especially risky.
However, I still find myself extremely CCS-curious when it comes to coal power in Asia, where the coal asset based is still frighteningly young. The average coal power plant in India is just a little more than 10 years old, which is aout 30 years younger than the average in the US. Even though India has the cheapest solar power in the world, it’s hard to believe that those youthful coal assets will be retired within the next 20 years, which makes CCS a much more compelling retrofit option.
Next, natural gas power generation:
The competitiveness of CCS in natural gas generation is more complicated. In regions with strong wind & solar resources, and the ability to accelerate electric transmission expansion, I think renewables ought to maintain a competitive edge. But in places with weaker renewable resources, where nuclear power is the fossil fuel alternative to beat, applying CCS to gas power generation could be relatively attractive.
Lastly, industrial heat:
This is the domain in which I think CCS has the best chance to compete with clean power alternatives. The relatively high efficiency of burning coal or gas to generate heat, rather than power, translates into fewer tons of CO2 emissions for every joule of energy consumed. Hence, renewables will have a tougher time competing outside of the places with the very best wind & solar resources. CCS probably has the best odds of success in large facilities like steel mills and cement plants, but could potentially be applied to any facility in which fossil fuel is burned to generate high temperature heat.
Summing up these competitive dynamics:
CCS could absolutely have a role to play in decarbonizing large industrial sites and power plants, especially in regions with tepid renewable resources. Of course, this depends on how much additional progress we make on the cost of renewables and nuclear energy. And, crucially, it also depends on the cost of CCS falling into the ranges estimated by the DOE.
What about Direct Air Capture?
Buildings, vehicles, and agriculture, combined, account for around a third of total global greenhouse gas emissions. Unfortunately, none of these sectors make good targets for point source CCS.
Okay, okay… Capturing carbon directly from building boilers and furnaces is certainly possible. So is capturing CO2 from vehicle tailpipes. But there are all kinds of practical infrastructure constraints and questions which make my head spin. How much would it cost to lay yet another set of pipes, in an urban environment, in order to gather up all the CO2 from these distributed sites.? How much would it cost to build a similar network running along highway corrridors, and snaking among urban gas stations? Alternatively, how many trucks capable of carrying liquified CO2 would you need to stop at these sites every week in order to deliver all of the captured carbon to a remote sequestration hub?
I’ll admit to being extremely skeptical that any of this can be done affordably.
In fact, for some of these small, disributed, and mobile sources of emissions, I’ve come to believe that it might be easier to capture CO2 from the ambient air than to capture it at the source.
I know this sounds a bit nuts! Recall from earlier in this post that CO2 constitutes just 0.04% of the molecules in the air, which is about a hundred times lower concentration than the CO2 emissions from any vehicle tailpipe or building flue. Hence, the thermodynamics of “direct air capture” are indeed daunting.
On the other hand… Fundamentally, the minimum energy requirement for direct air capture is roughly 2-3X the minimum requirement for point-source capture. So yes, DAC is a lot more energy-intensive than point source CCS, but that energy intensity might not be a deal-breaker.
Because CO2 mixes fairly readily in the earth’s atmosphere, removing carbon from the atmosphere at any spot on earth is roughly equivalent, from a climate standpoint, as doing so anywhere else. In a way, this makes DAC a remarkably elegant solution to our carbon problem, neatly separating the act of emitting carbon from the act of dealing with it. This makes DAC a uniquely flexible solution, both temporally and geographically, which gives DAC some conceptual advantages that are worth a closer look:
It can be theoretically deployed at ungodly scale, anywhere in the world. So, what DAC sacrifices in CO2 concentration, it can partially make up for in practically unlimited economies of scale.
DAC is theoretically capable of running on intermittent renewable power. Modest amounts of storage will probably be needed in order to ensure reasonably high utilization of the DAC machines, but my guess is somewhere around 8-10 hours of storage will be sufficient. There’s no need for truly firm power supply.
Crucially, DAC is the only surefire solution for dealing with carbon which has already been emitted. This will probably become very important in the decades to come, given that many climate models suggest we’ll probably need to start turning carbon-negative, in order to stave off the worst risks of climate change, at some point later this century.
DAC is also the only way to truly “recycle” carbon at scale. This makes it the only way to enable the continuous production and consumption of so-called “electrofuels” which are synthesized from atmospheric carbon and clean hydrogen. In my last post on “Moving the Big Heavy Things” I noted that this strategy is inherently a lot more expensive than simply continuing to burn fossil fuel while employing DAC to sequester the emissions. So if DAC exists, and sequestration is not too expensive, I’m not personally convinced that electrofuels make much sense. But some smart people are really into them!
Most importantly: DAC can be strategically deployed in the absolute most favorable locations worldwide, specifically those which sit at the intersection of:
Massive geologic sequestration potential
Ultra-strong renewable energy resources
And, very few neighbors
Building enormous DAC hubs in these sorts of places could theoretically enable carbon to be captured by the gigaton, while avoiding some of the critical bottlenecks which are currently bedeviling infrastructure buildout for both renewables and CCS alike. DAC has no need for long carbon pipelines or electric transmission lines, which hopefully means a lot fewer objections from NIMBYs.
Consider Montana and North Dakota.
These two states are blessed with vast amounts of underground pore space, in saline reservoirs, as well as tremendous untapped wind energy resources which are effectively stranded indefinitely, due to lack of transmission. Imagine thousands of wind turbines powering hundreds of DAC machines, pumping CO2 directly into the earth underneath.
I understand this might read a lot like science fiction. So, for the sake of making the concept more tangible, here’s a picture of a real-life DAC machine built by a company called Global Thermostat (which was recently acquired by Zero Carbon Systems).
This thing is a small pilot project, but given the ultra early stage of the industry it’s also one of the biggest DAC systems in the world. It’s capable of capturing about a thousand tons of CO2 per year, which is around 0.0000026% of global emissions. It uses a bunch of giant fans to blow air through a honeycomb structure coated in a solid “sorbent” material, which is a kind of alternative to the liquid amines I described earlier in this post. Clean electricity is required to power the fans, to periodically heat up the sorbent in order to make it let go of the CO2 that it’s captured, and to pump that CO2 underground.
Here’s an illustrative design for a plant which would capture a thousand times more carbon. (Note the tiny truck near the bottom of the image, for scale.)
It would take about than 6,400 of these plants in order to capture all of the CO2 emitted by the US each year. Powering each one would require hundreds of wind turbines, or thousands of acres of solar panels, or maybe a nuclear power plant. (Or, most likely, some combination of energy sources.)
So… I agree. This does sound nuts. But I also find myself wondering: Is it nuttier than building networks of sewage pipes underneath our cities and towns? Is it nuttier than digging landfill after landfill to absorb our endless stream of municipal solid waste?
Yes, probably so. But if you’ve learned anything from this series of big questions, I hope it’s that we need to get a little bit nutty in order to solve our global carbon problem.
Of course, all of this only works if DAC can be made a lot cheaper than it is today. So, the key question is: Can DAC ever be made affordable?
Theoretically, yes. My EIP colleague, Dr. Eve Hanson, has been studying this question for years. At the Rocky Mountain Institute, her analysis outlined a path to DAC-ing carbon out of the sky for less than $150 per ton. Remarkably, that’s right in line with higher-end estimates of the cost of point-source CCS. It’s amazing what freedom from the constraints of time and space can do…
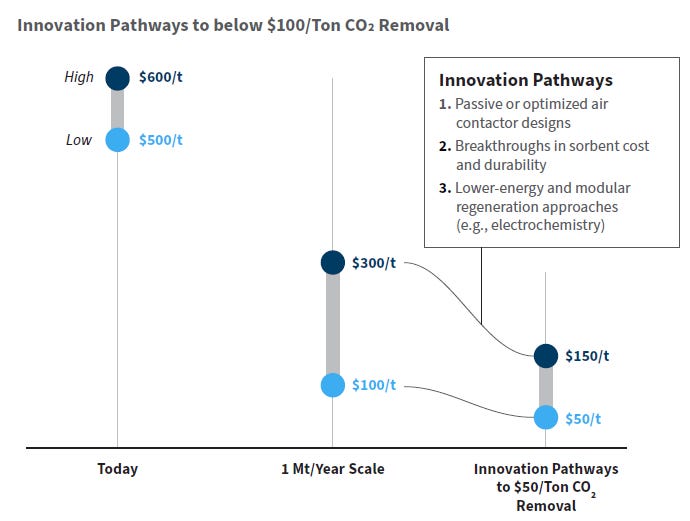
Personally, I’m convinced that this kind of technical path exists, but it’s proving to be one of the most difficult and uncertain paths amongst any of the major categories of “climate tech”. My personal view is that we don’t really need DAC, at least not for the next couple of decades. In the meantime, there are just too many hurdles to developing a robust market for the product DAC is selling. Until governments step into the domain of carbon removal in a major way, DAC will be too dependent on the largess of a small cadre of especially climate-forward buyers, like Microsoft and Stripe.5 It’s hard to see this market sustaining the kind of deployment pace that’s necessary to make material progress down the DAC cost curve.
So, will CCS make a lot of my other big questions moot?
I’ve now written more than 30,000 words attempting to answer what I view as the ten biggest questions in the field of energy and climate technology. So, I’m very pleased to say that the answer to this final question is “No”, because that means my first nine essays still matter!
So no, CCS won’t make all of my other questions moot. In fact, I’ve come to believe that the broad theoretical applicability of CCS and DAC mean the opposite is true: The answer to all of my other big questions will determine how much we come to rely on CCS.
Will we clear the path for a lot more electric transmission? If so, more regions will be able to harness low-cost renewables, which will tend to beat CCS as a solution for decarbonizing power generation. When will we get serious about nuclear? (or find something ‘nuclear-esque’?) If we launch a nuclear renaissance in the early 2030s, which I predict we will, then CCS will be faced with another major competitor. How will we move the big, heavy things? And how will we keep warm in the winter? The more buildings and vehicles we’re able to electrify, the less we’ll need to rely on DAC for offsetting continued fossil fuel combustion, or for producing sustainable electrofuels.
Hence, the fate of CCS is intertwined with nearly every big question in climate tech…. which is why I view the opportunity for CCS as one of the biggest uncertainties of them all.
…
As I close out this series, I’d like to add a final note to those of you who’ve been reading along, and encouraging me to write: THANK YOU. I hope you’ve enjoyed yourself, and have learned a thing or two along the way.
And for those of you who are new to Steel For Fuel, and like what you’ve read in this final post, here’s a link to the beginning of this series!
“$144.6 Billion All-Time High Local Spending on Water and Sewer Utilities in 2020 – CARES Act Fiscal Stimulus Makes an Impact”, US Conference of Mayors, 2022
According to the St Louis Federal Reserve’s FRED dataset, “Gross domestic Product: Waste management and remediation Services (562)”.
I’m not the first person to be taken with this analogy. At Energy Impact Partners, our Chief Technology Officer, Michael Webber has been using it for years to make the case for action on carbon emissions.
I say that EOR is “probably” not compatible with deep levels of decarbonization, because we will still continue to consume crude oil in most scenarios, at least as a chemical feedstock. And there are even some scenarios in which we continue to use limited amounts of oil-derived fuels which are offset by carbon removal (including DAC) elsewhere in the system.
The product DAC is selling: Engineered atmospheric carbon removal.
Have been following this series closely - terrific framing, and as a data person, even better analysis. Thanks!
It's ironic that this piece opens with the statement that " In many sectors, replacing fossil fuel while maintaining energy affordability & security is, I’m sorry to say, wickedly difficult." Because the rest of the piece describes an even more wickedly difficult solution through CCUS. At least with renewables, storage, and nuclear, we have known technologies that are operating at large scale, and have demonstrated periods of zero-carbon electricity in several regions of the world. Moreover, it's ironic that this piece was published on the day that we heard that renewable generation outpaced nuclear generation for the first time in the US - https://oilprice.com/Latest-Energy-News/World-News/US-Solar-and-Wind-Power-Generation-Tops-Nuclear-for-First-Time.html.
Finally, I'd recommend a review of Michael Barnard's thorough review of CCUS here - https://cleantechnica.com/2024/06/30/ccus-is-mostly-an-oil-gas-shell-game-sfu-seminar-slides-notes/. He makes the point that CCS simply cannot operate at the scale necessary to be material.